As the demand for renewable energy continues to rise, maximizing the efficiency of solar power generation has become a top priority. One of the critical components that enhance solar power system performance is the MPPT inverter. Maximum Power Point Tracking (MPPT) inverters optimize energy extraction from solar panels, ensuring higher efficiency and improved power output. In this comprehensive guide, we explore MPPT inverters, their working principles, benefits, types, and how they compare to conventional inverters.
Table of Contents
What is an MPPT Inverter?
An MPPT inverter is a device that combines a solar inverter with Maximum Power Point Tracking (MPPT) technology to optimize the energy output from photovoltaic (PV) panels. Its primary function is to convert the direct current (DC) electricity generated by solar panels into alternating current (AC) electricity suitable for use in homes or businesses, while simultaneously ensuring that the PV panels operate at their maximum power point.
How Does an MPPT Inverter Work?
Solar panels have a non-linear relationship between voltage and current, producing a unique power-voltage (P-V) curve that varies with sunlight intensity and temperature. The point on this curve where the product of current and voltage is highest is known as the Maximum Power Point (MPP). MPPT inverters employ sophisticated algorithms to locate and operate at this point, thereby extracting the maximum possible power from the PV array.
Step-by-Step Working Process
- Input from Solar Panels: Solar panels generate DC electricity with varying voltage and current based on sunlight and temperature.
- Voltage and Current Measurement: The MPPT inverter continuously measures the voltage and current output from the panels to calculate instantaneous power.
- Determining the Maximum Power Point (MPP): Using algorithms like Perturb and Observe or Incremental Conductance, the inverter identifies the optimal voltage and current combination where power output is maximized.
- Adjusting the Operating Point: Once the MPP is identified, the inverter adjusts the electrical operating point of the panels, often through a DC-DC converter, to maintain operation at this optimal point.
- DC to AC Conversion: The optimized DC power is then converted into AC power suitable for use in homes or businesses.
- Continuous Monitoring and Adjustment: The inverter perpetually monitors environmental conditions and panel outputs, making real-time adjustments to stay at the MPP as conditions change.
Benefits of Using MPPT Inverters
Higher Efficiency
MPPT inverters optimize the power output from solar panels by continuously adjusting to the panels’ optimal voltage and current levels. This dynamic tracking can boost system efficiency by up to 30%, ensuring maximum energy harvest even under varying environmental conditions.
Improved Performance in Low-Light Conditions
MPPT technology excels in adjusting to changes in sunlight intensity, allowing solar panels to maintain higher efficiency during cloudy days, early mornings, or late afternoons. This adaptability ensures a more consistent energy supply regardless of fluctuating light conditions.
Enhanced System Flexibility
MPPT inverters can manage multiple strings of solar panels with different orientations or shading patterns. This capability is particularly advantageous in installations where roof space is limited or uneven, as it allows for the optimization of each panel’s output independently, maximizing overall system performance.
Faster and More Efficient Battery Charging
In systems incorporating battery storage, MPPT inverters provide a steady, optimized charge to batteries, promoting faster charging times and extending battery lifespan. This efficient charging process ensures that stored energy is readily available when needed, enhancing the reliability of the power supply.
Better Handling of Partial Shading
MPPT inverters are adept at managing scenarios where some panels are partially shaded. By individually optimizing the performance of each panel or string, they minimize the impact of shading on the overall system output, ensuring that unshaded panels continue to operate at peak efficiency.
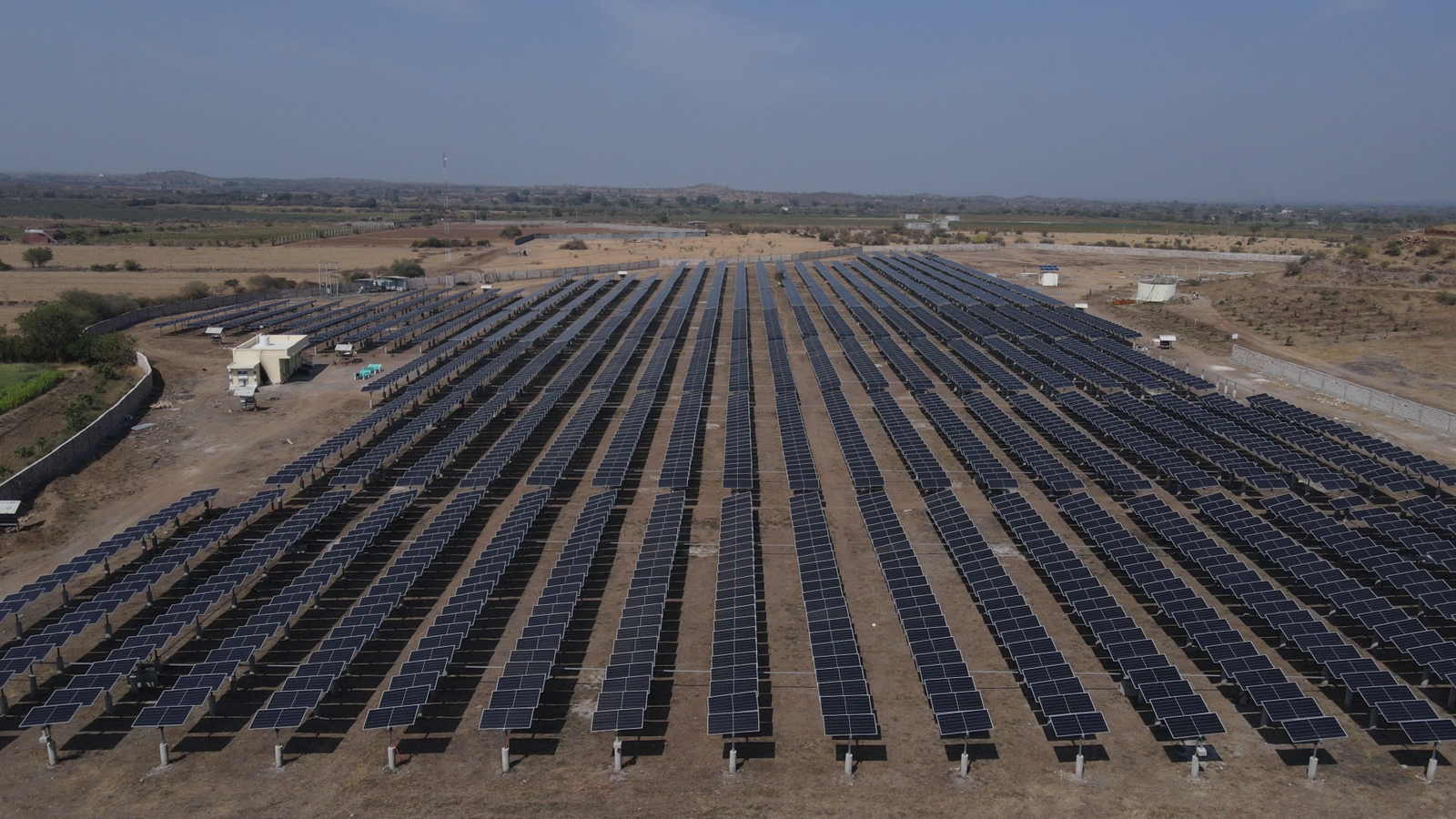
Types of MPPT Inverters
String MPPT Inverters
Single MPPT inverters are equipped with one Maximum Power Point Tracker. This means they monitor and optimize the power output of the entire PV array as a single unit. These inverters are most effective in installations where all solar panels have the same orientation and receive uniform sunlight without shading. In such scenarios, a single MPPT can efficiently manage the power output, ensuring optimal performance.
Micro MPPT Inverters
Microinverters are small inverters installed on each solar panel, with each unit incorporating its own MPPT. This setup allows for panel-level optimization, ensuring that the performance of one panel does not affect the others. Microinverters are particularly useful in installations with shading issues or where panels are installed in multiple orientations. They also simplify system expansion, as additional panels can be added without affecting the existing setup.
Hybrid MPPT Inverters
Hybrid MPPT inverters, also known as battery backup inverters, are designed to manage energy from both solar panels and battery storage systems. They can operate in grid-tied mode, feeding excess energy back to the utility grid, or in off-grid mode, supplying power directly to the load from batteries during grid outages. This versatility ensures a continuous power supply and enhances energy independence. Hybrid inverters are particularly beneficial in areas with unstable grids or for users aiming to maximize self-consumption of solar energy.
Dual MPPT Inverters
Dual MPPT inverters feature two independent trackers, allowing them to manage two separate strings of solar panels. This design is particularly advantageous for installations where panels are installed on different roof facets with varying orientations or are subject to partial shading. Each MPPT operates independently, optimizing the performance of its respective string and thereby enhancing the overall energy harvest of the system. This flexibility makes dual MPPT inverters a popular choice for residential and commercial applications with complex layouts.
Multiple MPPT Inverters
Expanding beyond dual configurations, multiple MPPT inverters come equipped with more than two trackers, sometimes managing up to four or more strings independently. These inverters are ideal for large-scale installations with diverse panel orientations and varying shading conditions. By optimizing each string separately, multiple MPPT inverters maximize the overall system efficiency and energy production, making them suitable for complex commercial and industrial solar projects.
MPPT Inverter vs. PWM Inverter
Feature | MPPT Inverter | PWM Inverter |
---|
Efficiency | 25-30% higher efficiency | Less efficient, energy loss |
Voltage Matching | Works with higher voltage panels | Requires panel voltage to match battery |
Performance in Low Sunlight | Optimized energy extraction | Reduced performance |
Cost | More expensive | More affordable |
Suitability | Best for large solar systems | Suitable for small-scale applications |
Energy Utilization | Maximizes power output | Wastes excess power as heat |
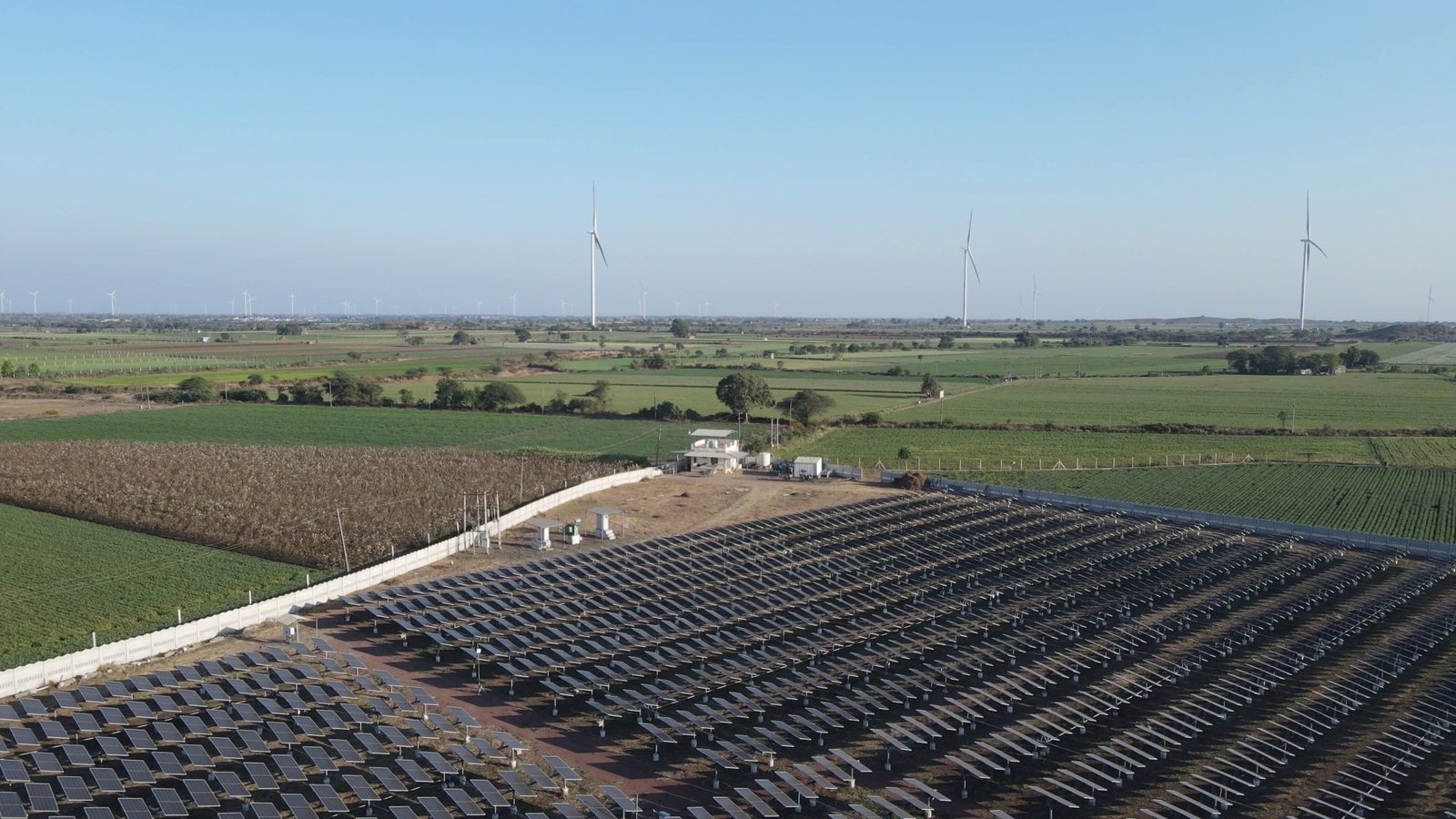
How to Choose the Best MPPT Inverter
Selecting the right Maximum Power Point Tracking (MPPT) inverter is crucial for optimizing the performance and efficiency of your solar energy system. Here’s a comprehensive guide to help you make an informed decision:
1. Determine Your System’s Power Requirements
- Total Power Output: Calculate the combined wattage of your solar panels. Ensure the MPPT inverter can handle at least this amount, preferably with some additional capacity to accommodate potential increases in sunlight intensity.
2. Ensure Voltage Compatibility
- System Voltage: Confirm that the inverter’s input voltage range aligns with your solar array’s output voltage.
- Battery Bank Voltage: If your system includes battery storage, ensure the inverter is compatible with your battery bank’s voltage (e.g., 12V, 24V, 48V).
3. Evaluate the Number of MPPT Channels
- Single vs. Multiple MPPTs: For installations with panels facing different directions or experiencing varying shading, inverters with multiple MPPT channels can optimize each string’s performance independently.
4. Assess Efficiency Ratings
- Conversion Efficiency: Look for inverters with high efficiency ratings (typically above 95%) to maximize energy harvest.
5. Consider Environmental Factors
- Temperature Range: Ensure the inverter operates effectively within your local temperature extremes.
- Ingress Protection (IP) Rating: For outdoor installations, select inverters with appropriate IP ratings to withstand environmental conditions.
6. Review Safety and Compliance Features
- Protective Mechanisms: Opt for inverters equipped with safeguards against overloads, short circuits, and reverse polarity.
- Certifications: Ensure the inverter meets relevant international and local standards.
7. Examine Monitoring and Connectivity Options
- Data Monitoring: Inverters with built-in monitoring allow you to track performance and identify issues promptly.
- Connectivity: Features like Wi-Fi or Bluetooth enable remote monitoring and integration with smart home systems.
8. Plan for Future Expansion
- Scalability: If you anticipate expanding your solar array, choose an inverter that can accommodate additional panels without requiring a complete system overhaul.
9. Evaluate Manufacturer Support and Warranty
- Warranty Period: Longer warranties often indicate confidence in product durability.
- Customer Support: Responsive and accessible support can be invaluable for troubleshooting and maintenance.
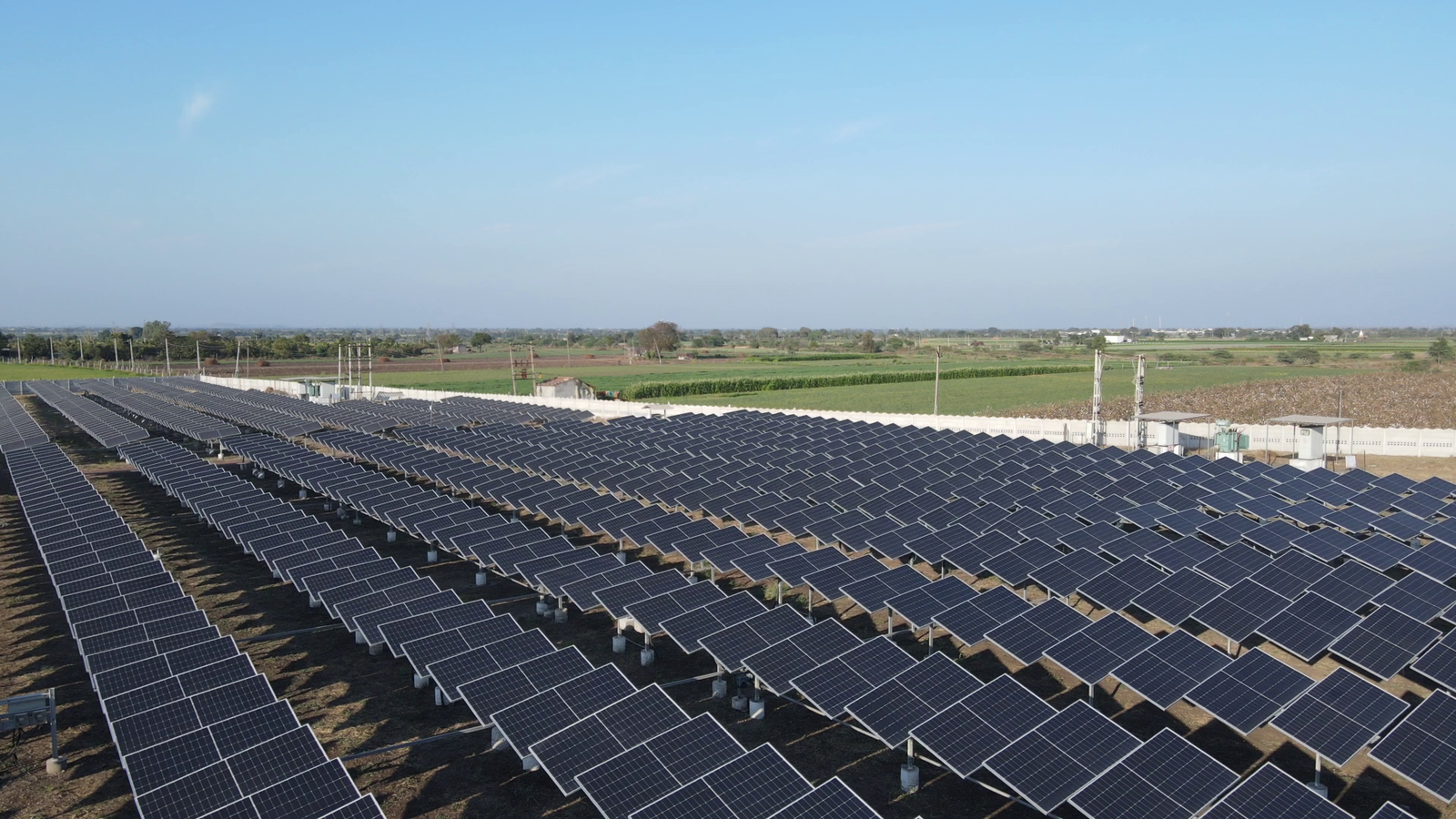
Installation and Maintenance of MPPT Inverters
Installing and maintaining an MPPT (Maximum Power Point Tracking) inverter is essential to ensure the efficiency, longevity, and safety of your solar power system. A well-installed and properly maintained inverter maximizes energy conversion and prevents potential breakdowns. This guide covers the step-by-step installation process, essential maintenance tips, and troubleshooting techniques to keep your MPPT inverter in top condition.
Step-by-Step Installation of an MPPT Inverters
1. Pre-Installation Preparations
Before installing the MPPT inverter, make sure you have:
- A suitable location (well-ventilated, shaded, and free from moisture).
- The correct inverter model is compatible with your solar panel and battery system.
- All necessary tools (screwdrivers, wiring, fuses, safety gloves, etc.).
- A circuit breaker or fuse box for protection.
2. Wiring the Solar Panels to the MPPT Inverter
- Check the Voltage – Ensure your solar panel output matches the inverter’s voltage input range.
- Series or Parallel Connection – Connect panels in series to increase voltage or in parallel to increase current.
- Use Proper DC Connectors – Securely connect the positive (+) and negative (-) terminals to avoid reverse polarity.
3. Connecting the MPPT Inverter to the Battery (If Applicable)
- Match Battery Voltage – Ensure the battery bank voltage is compatible with the inverter.
- Use Correct Cable Size – Thick cables prevent voltage drops and overheating.
- Install a Fuse – Protects the system from electrical surges.
4. AC Wiring for Grid-Tied Systems
- Connect to the Main Breaker Panel – Ensure proper grounding to prevent electrical hazards.
- Check for Compliance – Follow local electrical codes and regulations.
- Test the System – Once all connections are secure, test the inverter’s output and check for any error codes.
Routine Maintenance for MPPT Inverters
To maintain optimal performance, regular maintenance of your MPPT inverter is crucial. Here are the key steps:
1. Regular Cleaning and Inspection
- Dust and Dirt Removal – Wipe the inverter casing and vents to prevent overheating.
- Check Wiring and Connections – Ensure all cables are tightly secured and show no signs of wear.
- Inspect Cooling Fans – Ensure the fans are functioning properly to prevent overheating.
2. Monitoring Performance Metrics
- Check the LCD Display/App – Most MPPT inverters provide real-time data on power generation.
- Look for Error Codes – Address warnings or system failures promptly.
- Monitor Battery Performance – If using batteries, check their charging and discharging cycles.
3. Preventive Measures for Longevity
- Ensure Proper Ventilation – Avoid placing the inverter in enclosed or overheated spaces.
- Keep Away from Moisture and Direct Sunlight – Protect from environmental damage.
- Perform a Full System Check Annually – Engage a professional for a thorough inspection.
Future of MPPT Inverters
The future of Maximum Power Point Tracking (MPPT) inverters is set to see significant advancements due to the growing demand for efficient solar energy solutions. Key trends include the integration of Artificial Intelligence and Machine Learning, which enable predictive maintenance and system optimization, and seamless integration with energy storage systems. Smart grid compatibility is also becoming a critical feature, with inverters equipped with advanced grid support functionalities. Advanced Maximum Power Point Tracking algorithms optimize power output, even under challenging conditions. Modular and scalable architectures are being adopted, allowing for flexible system expansion and easy integration with additional components. Environmental sustainability is also a key focus, with manufacturers adopting eco-friendly materials and energy-efficient designs.
Conclusion
MPPT inverters are a game-changer in solar energy systems, ensuring maximum power extraction from photovoltaic panels. By continuously tracking and adjusting to the optimal voltage and current, these inverters significantly enhance efficiency, reduce energy losses, and improve system reliability. Whether you are setting up a residential solar system, a large-scale commercial installation, or an off-grid power solution, choosing the right type of MPPT inverter is crucial for achieving optimal performance.
With advancements in solar technology, MPPT inverters continue to evolve, offering better efficiency, enhanced monitoring capabilities, and seamless integration with battery storage solutions. Investing in a high-quality MPPT inverter is a step toward sustainable energy consumption, reducing dependence on conventional power sources while maximizing savings.
Are you ready to upgrade your solar system with a high-efficiency MPPT inverter? At Soleos Solar, we offer premium MPPT inverters tailored to your energy needs. Contact us today for expert guidance and the best solar solutions for your home or business.